Neogene Snake River Plain-Yellowstone volcanic province
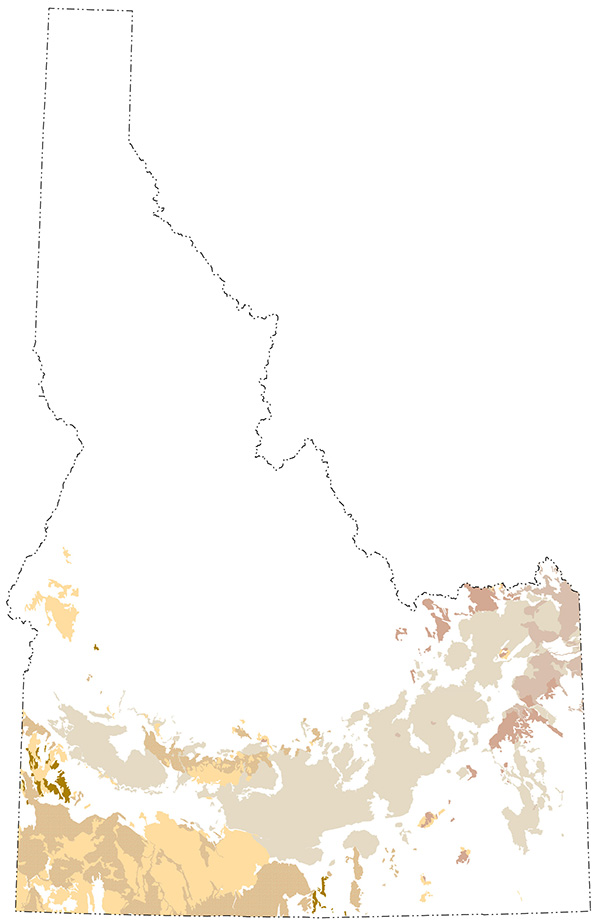
(Above) Exposures of Miocene and younger volcanic rocks.
Yellowstone National Park sits above what is often referred to as the Yellowstone Caldera or Yellowstone Plateau, which is interpreted by most researchers to be sitting above a stationary mantle plume or “hotspot." The eastern Snake River Plain is thought to be a result of passage of the North American Plate over that hotspot and hence can be thought of as the ancient track of the Yellowstone hotspot (e.g., Pierce and Morgan, 2009).
PDF slideshows: Snake River Plain and Snake River Plain topographic development by Paul Link and Snake River Plain-Yellowstone volcanism by Scott Hughes.
Neogene Snake River Plain
The Snake River Plain is a broad arcuate topographic depression that extends across southern Idaho. The western Snake River Plain sits in a fault-bounded graben whereas the eastern Snake River Plain (ESRP) is a large structural downwarp that formed due to the weight of mafic magmas in the subsurface as well as overlying volcanic rocks. The Owyhee Plateau is genetically related to the Snake River Plain, although it has remained a highland region.
Hotspot volcanism commenced ~17 Ma in northeastern Nevada and continues to the present. Ignimbrites and tuff deposits marginal to the Snake River Plain record the path of the movement of the North American Plate over the stationary hotspot at approximately 2.35 cm/year (Anders et al., 2014). Basaltic lava fields, polygenetic eruptive centers, and rhyolite domes that spatially and temporally overlie Yellowstone Hotspot volcanism constitute the upper 1-2 km of ESRP stratigraphy. Many of the basalts of the ESRP erupted along volcanic rift zones (VRZ) that are oriented parallel to the direction of regional Basin and Range extension.
Current silicic volcanic activity is centered in the Yellowstone area. Previous silicic volcanic centers likely produced a topographic bulge that coincided with the continental divide. As the North American plate migrated to the southeast and volcanic activity shifted to new regions, the extinct calderas gradually subsided to their present elevations due to thermal and gravitational effects.
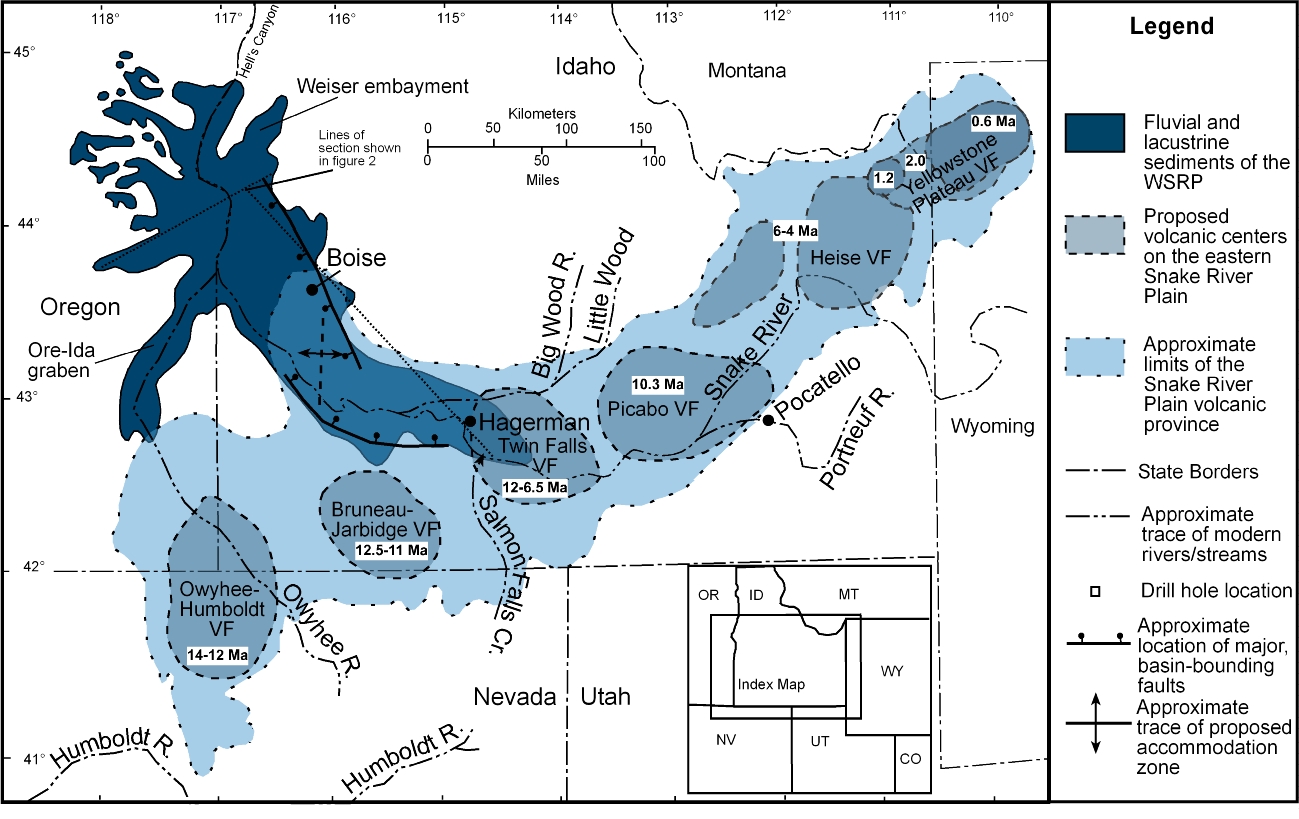
(Above) Map showing the northeastward apparent motion of hotspot migration and the ages of the various calderas. From Link and Phoenix (1996), as simplified from Pierce and Morgan (1992).
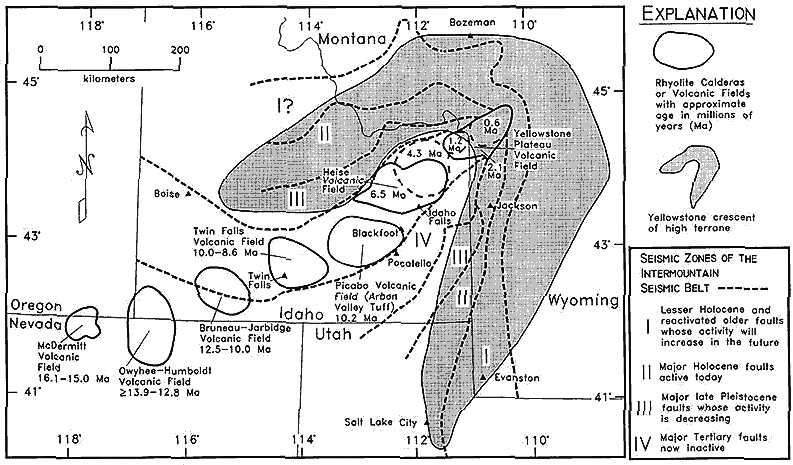
(Left/Above) Four zones of seismic activity are associated with the migration of the hotspot (Figure 4 after Pierce and Morgan, 1992). Zone II contains active Holocene faults that are thought to be connected with current volcanic activity of the Yellowstone Hotspot. Zone III contains late Pleistocene faults that are decreasing in activity. Zone IV contains faults that are no longer active. The zones form belts that curve around the hotspot track.
Geology of the Snake River Plain
The western and eastern Snake River Plains are topographically continuous and seem similar; however, they are structurally quite different. The western Snake River Plain (WSRP) is a NW trending graben; both the land surface and the rock layers dip towards the axis of the plain (Shervais, et al., 2005; Bonnichsen and Godchaux, 2002). The rocks that occupy the WSRP are rhyolitic tuffs and ash flows of the Idavada Volcanic Group (15 to 11 Ma in age), and fluvial and lacustrine sediments with interbedded basalt flows of the Idaho Group (Pierce and Morgan, 1992; Bonnichsen and Godchaux, 2002). Lake Idaho occupied the WSRP during the Pliocene epoch, as the WSRP subsided and the hotspot continued to the northeast (see Figure 2).
The eastern Snake River Plain is underlain by silicic and mafic volcanic rocks with local interbeds of continental sediments. Quaternary basalt flows cover ~95% of the surface of the ESRP (Kuntz et al., 1992). The Idavada silicic volcanics of the ESRP are lithologically similar to those of the WSRP but are younger in age (10 Ma to 6.2 Ma). The tuffs at Yellowstone (0.6 to 2 Ma) represent the youngest pulse of silicic volcanic activity associated with the hotspot (Pierce and Morgan, 1992).
(Above) Typical cross-section through volcanic rocks on the eastern Snake River Plain. Figure from Hughes et al., 1999.
There is no evidence of faulting along the margins of the ESRP even though the Basin and Range province borders its northern and southern margins. Basin and Range faults are oriented perpendicular to the axis the eastern Snake River Plain (Rodgers et al., 1990).
(Right/Below) Axis perpendicular to the Snake River Plain from Bestland 1998. Basaltic lava flows within the ESRP erupted from northwest-trending volcanic rift zones, which are oriented parallel to Basin and Range normal faults beyond the margins of the ESRP. These volcanic rift zones do not appear to connect to Basin and Range faults marginal to the plain (see Figure 7). Regional extension across the plain allowed for the propagation of mafic dikes from the middle and lower crust. (Kuntz et al., 1992; Hughes et al., 2002).
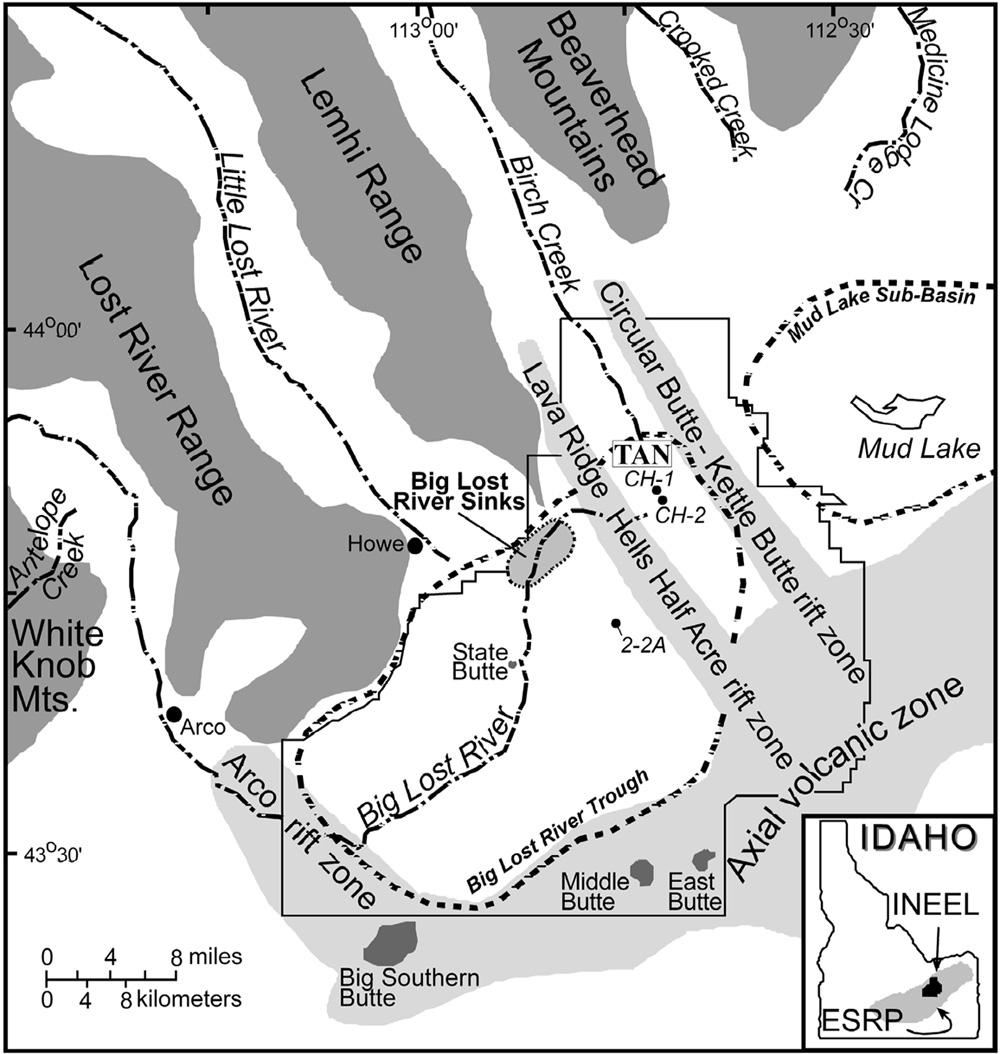
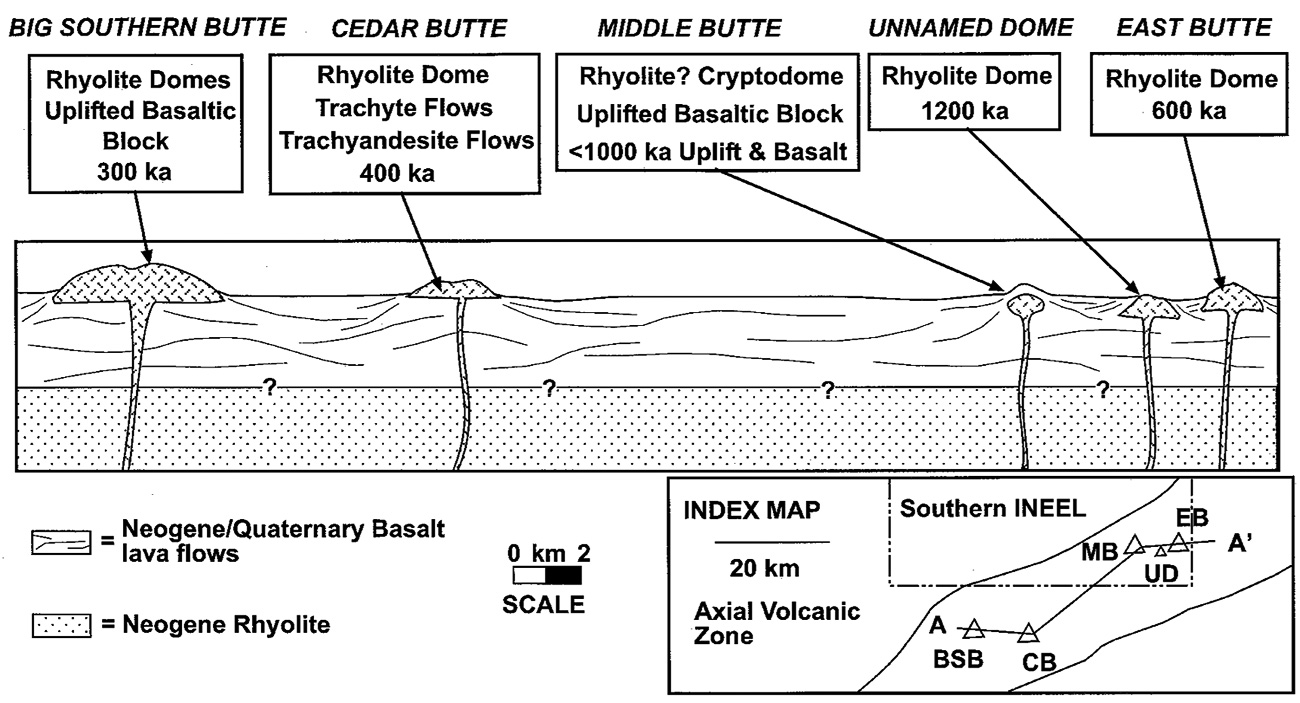
(Left/Above) Relative locations of the five major rhyolite domes ("buttes") on the ESRP (from Hughes et al., 1999). From south to north, these are Big Southern Butte, Cedar Butte, Middle Butte, Unnamed Butte, and East Butte. They are located near the extinct Picabo and Heise volcanic centers but are not directly associated with Yellowstone hotspot volcanism.
Evidence for a hotspot origin of the Snake River Plain The hotspot origin model of the Snake River Plain is the com
The hotspot origin model of the Snake River Plain is the commonly accepted model. Pierce and Morgan (1992; 2009) summarized several lines of evidence that support the hotspot model. The first line of evidence is the voluminous phase of mafic volcanism (Columbia River basalts) associated with the earliest apparent phase of hotspot activity. This was followed by a time transgressive record of silicic volcanism interpreted to be the result of the movement of the North American Plate over a stationary mantle plume (e.g., Anders et al., 2014).
A second line of evidence is the presence of a topographically high region centered near the Yellowstone caldera, called the Yellowstone crescent of high terrain. This crescent of high terrain flares out in a bow wave-like pattern about the volcanic track and heads in front of the volcanic progression and favors a sub-lithosphere driver. As the hotspot migrated to the northeast with respect to the North American Plate, the highlands subsided due to cooling of the underlying crust and crustal loading from the eruption of post-hotspot basalts. This changed drainage patterns in river systems within and adjacent to the ESRP.
The location of the Continental Divide locally corresponds to the migration of the hotspot. The topographic high produced by the hotspot produced radial drainage systems that flowed away from the volcanic center. In the Miocene epoch, when Snake River Plain volcanism began, the continental divide was located west of its current position and local streams drained toward the Atlantic Ocean; sediments were transported eastward, northward and southward away from the location of the volcanic high (Beranek et al., 2006).
(Above) Topographic and drainage evolution of the ESRP-Yellowstone system (modified from Link et al., 1999 and Beranek et al., 2006).
As the continental divide moved eastward across southern Idaho, rivers south of the Snake River Plain began to flow south and east. Subsidence in the wake of hotspot migration caused a drop in the base level of the Snake River Plain which initiated headward erosion toward the modern Snake River channel. This eventually led to stream capture and the shift of drainage to the Pacific Ocean.
An additional line of evidence is the apparent match in timing of high rates of Basin and Range faulting and the locations of silicic volcanism (Rodgers et al., 2002). This suggests that weakening of the lithosphere during heating led to enhanced rates of faulting. The four zones of increasing seismic activity form the Intermountain seismic belt around the current location of hotspot activity.
Further information about the Yellowstone-eastern Snake River Plain can be found in the Digital Atlas of Idaho: Snake River Plain page and Rocks, Rails, and Trails page.
Continue to Module 12 - Pleistocene glaciation
References and further reading
Beranek, L.P., Link, P.K., Fanning, C.M., 2006, Miocene to Holocene Landscape Evolution of the Western Snake River Plain Region, Idaho: Using the SHRIMP detrital zircon provenance record to track eastward migration of the Yellowstone Hotspot. Geological Society of America Bulletin, September/October 2006, p. 1027-1050.
Bonnichsen, B., and Godchaux, M.M., 2002, Late Miocene, Pliocene, and Pleistocene Geology of Southwestern Idaho With Emphasis on Basalts in the Bruneau-Jarbidge, Twin Falls, and Western Snake River Plain Regions, in Bill Bonnichsen, C.M. White, and Michael McCurry, eds., Tectonic and Magmatic Evolution of the Snake River Plain Volcanic Province: Idaho Geological Survey Bulletin 30, p. 233-312.
Hackett, W.R. and Smith, R.P., 1992, Quaternary volcanism, tectonics, and sedimentation in the Idaho National Engineering Laboratory area, in Wilson, J.R., ed., Field Guide to Geologic Excursions in Utah and Adjacent areas of Nevada, Idaho, and Wyoming: Utah Geological Survey, Miscellaneous Publication 92-3, p. 1-18.
Hughes, S. S., Smith, R. P., Hackett, W. R., and Anderson, S. R., 1999, Mafic Volcanism and Environmental Geology of the Eastern Snake River Plain, Idaho, in Hughes, S. S. and Thackray, G. D., eds., Guidebook to the Geology of Eastern Idaho: Idaho Museum of Natural History, p. 143-168.
Hughes, S.S., Wetmore, P.H., Casper , J.L., 2002, Evolution of Quaternary Tholeiitic Basalt Eruptive Centers on the Eastern Snake River Plain, Idaho, in Bonnichsen, B., White, C.M., and McCurry, M., eds., Tectonic and Magmatic Evolution of the Snake River Plain Volcanic Province: Idaho Geological Survey Bulletin 30, p. 363-385.
Kuntz, M.A., Champion, D.E., Spiker, E.C., Lefebvre, R.H., and McBroome, L.A., 1982, The Great Rift and the evolution of the Craters of the Moon lava field, Idaho, in Bonnichsen, B. and Breckenridge, R.M., eds., Cenozoic Geology of Idaho: Idaho Bureau of Mines and Geology Bulletin 26, p. 423 - 438.
Kuntz, M.A., Covington, H. R., and Schorr, L. J., 1992, An overview of basaltic volcanism of the eastern Snake River Plain, Idaho, in Link, P. K., Kuntz, M. A., and Platt, L. P., eds., Regional geology of eastern Idaho and western Wyoming: Geological Society of America Memoir 179, p. 227-267.
Link, P.K., Fanning, C.M., Beranek, L.P., 2005. Reliability and longitudinal change of detrital-zircon age spectra in the Snake River system, Idaho and Wyoming: An example of reproducing the bumpy barcode. Sedimentary Geology 182, 101-142.
Link, P.K., Reading, R.W., Godfrey, A.E., and Prevedel, D., 1999, Topographic development of the Snake River Plain, Idaho, in Hughes, S.S., and Thackray, G.D., eds., Guidebook to the geology of eastern Idaho: Pocatello, Idaho, Idaho Museum of Natural History, back cover.
Link, P.K. and Phoenix , E.C., 1996, Rocks Rails & Trails, 2nd Edition: Idaho Museum of Natural History, 194 p.
Pierce, K. L. and Morgan, L. A., 1992, The track of the Yellowstone hot spot: Volcanism, faulting, and uplift, in Link, P. K., Kuntz, M. A., and Platt, L. B., eds., Regional Geology of Eastern Idaho and Western Wyoming: Geological Society of America Memoir 179, p. 1-53.
Rubin, A.M. and Pollard, D.D., 1987, Origins of blade-like dikes in volcanic rift zones: U.S. Geological Survey Professional Paper 1350, Chapter 53, p. 1449-1470.
Shervais, J.W, Kauffman, J.D., Gillerman, V.S., Othberg, K.L., Vetter, S.K., Hobson, V.R., Zarnetske, M., Cooke, M.F., Mathews, S.H., and Hanan, B.B., 2005, Basaltic Volcanism of the Central and Western Snake River Plain: A Guide to Field Relations Between Twin Falls and Mountain Home, Idaho, in Pederson, J., and Dehler, C.M., eds., Interior Western United States: Geological Society of America Field Guide 6, 26 p.
Credits
This page was originally created by Paul Link, Idaho State University; it was modified and updated by Dave Pearson at Idaho State University.