Cenozoic crustal extension
Idaho was the site of major crustal extension during two times in the Cenozoic period: (1) Eocene crustal collapse during Trans-Challis faulting, which was accompanied by formation of Cordilleran metamorphic core complexes; and (2) widespread Miocene to Present continental extension that characterizes the Basin and Range Province.
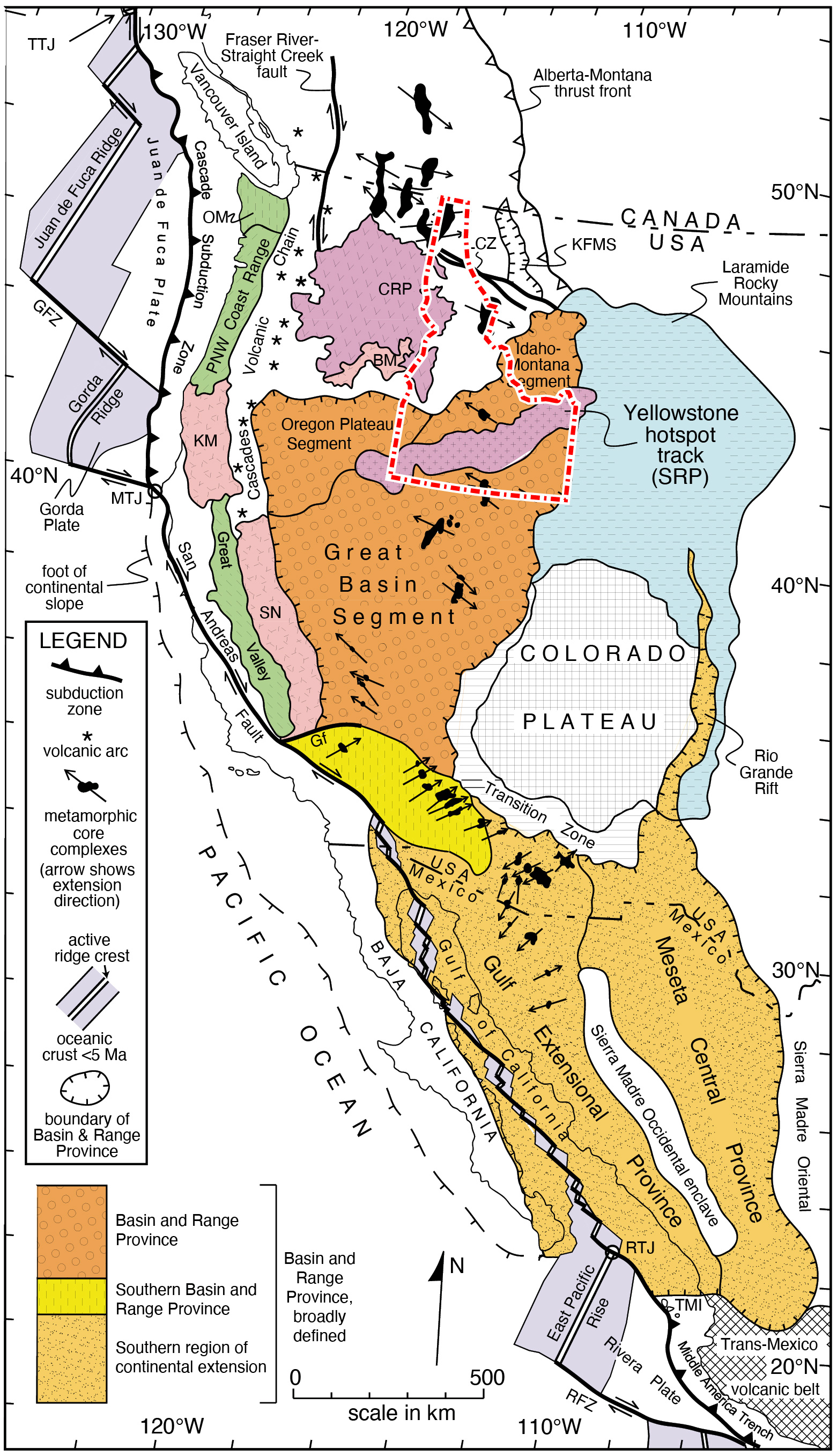
(Left/Above) Position of the Basin and Range Province in the western Cordillera. Modern triple plate junctions: MTJ—Mendocino; RTJ—Rivera; TTJ—Tofino. Other abbreviations: BM—Blue Mountains; CRP—Columbia River Plateau (check pattern and red color denote extent of Columbia River Basalt lavas); KFMS—Kisenehn-Flathead-Mission-Swan extensional Paleogene basins; KM—Klamath Mountains; LCZ—Lewis and Clark fault zone; PNW—Pacific Northwest; RFZ— Rivera Fracture Zone; SN—Sierra Nevada; SRP—Snake River Plain; TMI—Tres Marias Islands (cross pattern and red color denote extent of bimodal volcanic suite). (modified from Dickinson, 2006).
The early phase of extension resulted in formation of low-angle normal faults with high extension magnitudes (>15 km), which brought metamorphic rocks up to the surface. Crustal extension during this phase began in Eocene time in southern British Columbia and Idaho, and migrated southward into Miocene and younger times in the southwestern U.S.
The more recent phase of extension is generally Miocene and younger and characterizes the Basin and Range Province, which includes most of eastern California, eastern Oregon, eastern Washington, Nevada, western Utah, southern and western Arizona, southeastern and east-central Idaho, westernmost Wyoming, and southwestern Montana. The Basin and Range Province is characterized by active extension, ranging from a total of 50% or less to 200% extension of the pre-Basin and Range land area (Faulds and Varga, 1998). This extension occurs mostly along large normal faults.
PDF slideshows: Borah-earthquake by Paul Link
Two phases of Cenozoic extension
At ~52 Ma, following the Sevier-Laramide orogeny, western North America was the site of a long mountain belt--the North American Cordillera--with thick, hot crust. During continued eastward subduction of remnants of the Farallon-Kula plates, mid-ocean ridges began to intersect the subduction zone, resulting in a transition from a subduction zone to a transform plate boundary. First, the ancestral Queen Charlotte transform plate boundary is thought to have developed; this major strike-slip fault system is now located outboard of western British Columbia. Later, the ancestral San Andreas transform boundary formed in the southwestern U.S.
First phase of Cenozoic extension: Metamorphic core complexes of the northern Rocky Mountains
Though the hot, overthickened crust of the North American Cordillera first began to gravitationally collapse in Late Cretaceous time--likely in a similar way to in the Tibetan Plateau and other large, convergent orogens (e.g., Dewey, 1988)--major crustal extension is thought to have begun in Eocene-Oligocene time in a region of very thick crust from southern British Columbia to central Idaho. This early phase of extension occurred at approximately the same time as development of the northern Queen Charlotte transform plate boundary and was concurrent with Challis magmatism and development of the northeast-trending Trans-Challis fault system. Some of the major normal faults formed during Eocene time in Idaho accommodated major horizontal extension, resulting in displacement of mid-crustal, basement rocks to the Earth's surface. In areas where the footwalls of these normal faults brought up metamorphic rocks from the middle crust and juxtaposed them against hanging walls of shallow crustal sedimentary and/or volcanic rocks, these fault systems are called "metamorphic core complexes." Metamorphic core complexes are also distinctive in that they often expose relatively shallowly dipping normal faults. Because most normal faults generally are steeply dipping, these low-angle normal faults have been the subject of research and controversy. Most workers agree that low-angle normal faults within metamorphic core complexes were originally more steeply dipping near the Earth's surface and were rotated to shallower dip angles, with continued slip rooting into shallowly dipping normal faults at deeper crustal levels.
(Above) One model for development of low-angle normal fault systems within metamorphic core complexes (modified from Kapp et al., 2008).
Eocene metamorphic core complexes exposed in Idaho include the Priest River metamorphic core complex, the Clearwater metamorphic core complex, the Bitterroot metamorphic core complex, and the Pioneer metamorphic core complex. The Albion-Grouse Creek-Raft River metamorphic core complex in southern Idaho was active in Oligocene time and then again in Miocene time during Basin and Range extension. The Pioneer metamorphic core complex in central Idaho was exhumed in the footwall of the Wildhorse detachment fault and exposes a complex suite of Archean and Proterozoic gneisses correlated with the Grouse Creek basement block. For more information about basement provinces in Idaho, see the basement module.
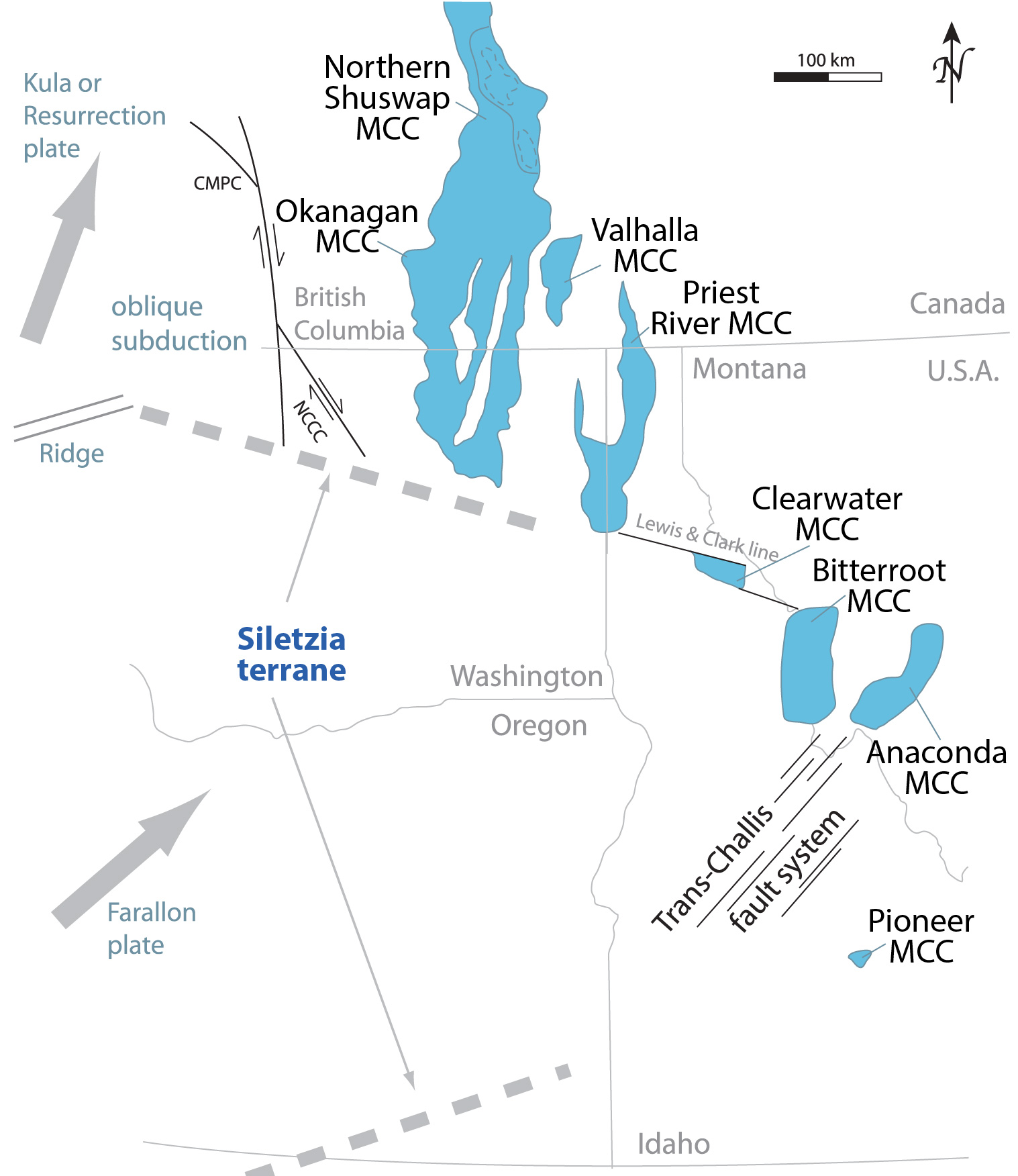
(Above) Hypothesized Eocene regional tectonic setting with early metamorphic core complexes shown (modified from Vogl et al., 2012).
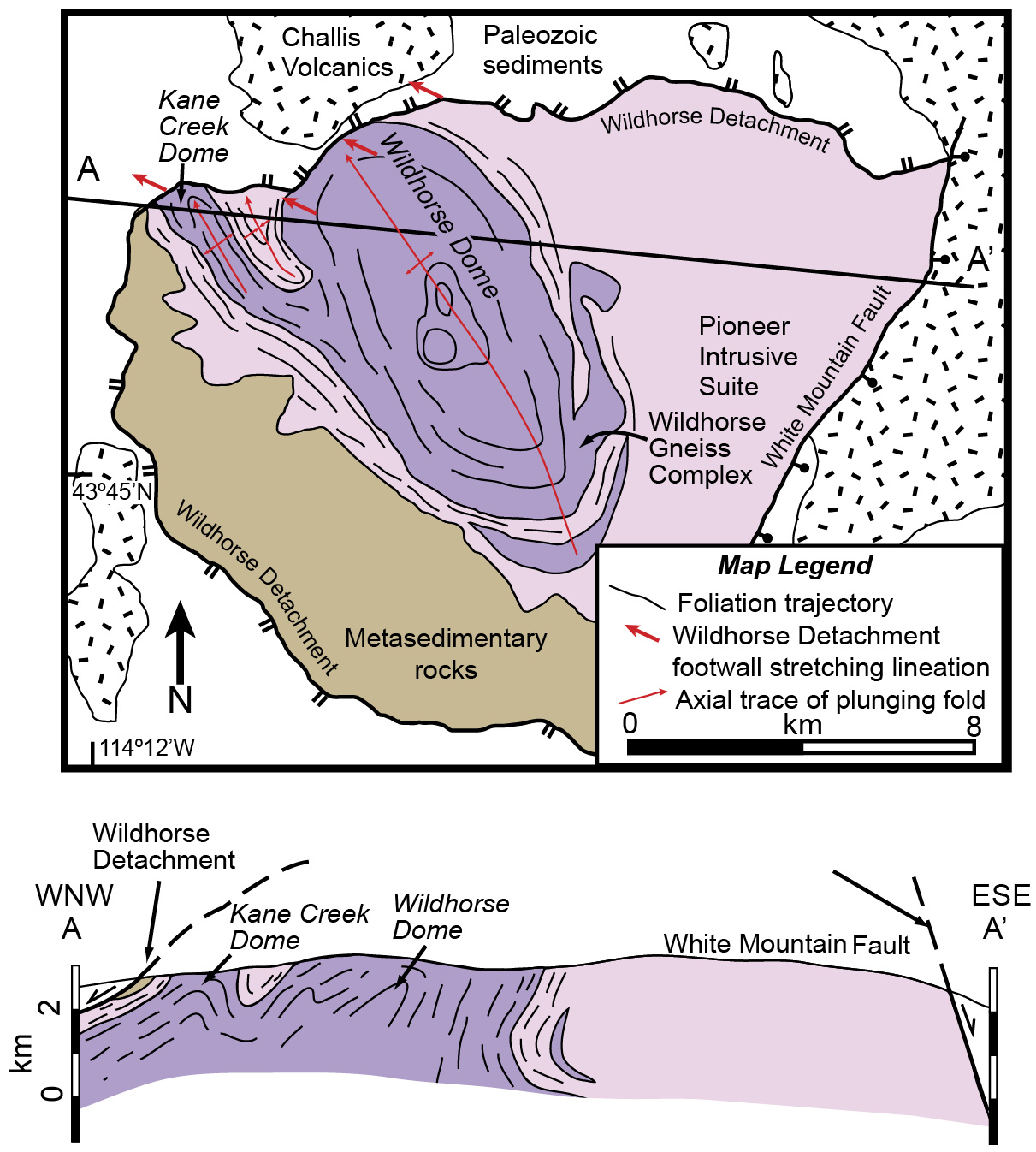
(Above) Generalized geologic map of the Pioneer metamorphic core complex in central Idaho (modified from McFadden et al., 2015).
Second phase of Cenozoic extension: Basin and Range
Basin and Range extension began during the Miocene Epoch (~17 Ma) and has continued through present, with the area of active extension propagating westward toward the Sierra Nevada, eastward into southeastern Idaho and westernmost Wyoming, and northward into the Yellowstone-southwestern Montana-east-central Idaho region. Crustal extension is a result of the transition from the older subduction zone to a more recent transform plate boundary, resulting in collapse of overthickened crust left over from the Sevier-Laramide orogeny. In eastern Idaho, Miocene and younger crustal extension within the Basin and Range Province was likely enhanced by crustal weakening associated with Yellowstone volcanism.
(Above) Time panels showing the evolution of the Pacific – North American transform boundary since ~20 Ma. Double red lines are mid-ocean ridges. MTJ, Mendocino triple junction; RTJ, Rivera triple junction. Dark orange areas denote areas of extension within the Basin and Range province. Panel F shows projected future development of the Pacific – North American transform, whereby the northward propagating Mendocino triple junction and Walker Lane – eastern California shear zone collide off the southern Oregon coast. (modified from Faulds and Henry, 2008).
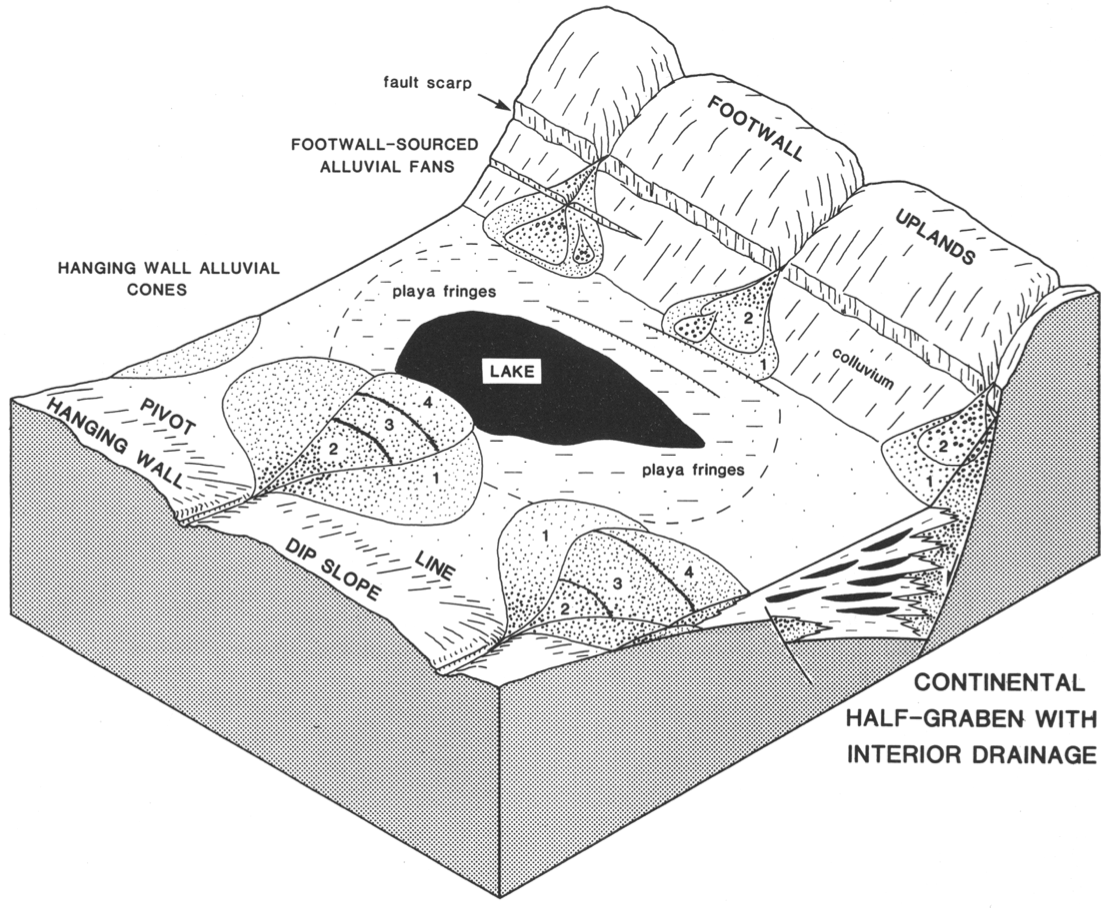
(Left/Above) Schematic perspective view of a typical half-graben fault system and hanging wall basin. Many of the Basin and Range faults in western North America have a similar geometry (from Leeder and Gawthorpe, 1987).
The most classic fault geometry within the Basin and Range province consists of steeply dipping (~60°) normal faults that shallow their dip angles with depths, a fault geometry called "listric." The Basin and Range province gets its name from the characteristic basins and ranges that occur next to each other, within half graben or horst-graben geometries. Down-dropping of normal fault hanging walls during major horizontal extension has resulted in low elevations and diversion of river systems into these depressions, often forming internally drained basins such as within the Great Basin.
Relationship of Basin and Range extension to transform plate boundary
Though the primary force driving Basin and Range extension is the gravitational potential energy remaining from thickened crust after the Sevier-Laramide orogeny, the Basin and Range Province is also linked to oblique continental rifting in the Gulf of California and accommodates up to 25% of the right lateral transform plate motion between the Pacific and North American plates (Atwater, 1970; Bennett et al., 2003). Within the western Basin and Range province in eastern California and northwestern Nevada, the Walker Lane and Eastern California shear zone show a mix of crustal extension and right-lateral strike-slip faulting that is the same orientation and sense as the San Andreas fault system. In the eastern Basin and Range Province, including in eastern Idaho, faults accommodate less strike-slip displacement and primarily accommodate east-west extension (Bennett et al., 2003).
(Right/Below) Regional map showing crustal extension direction constrained using global positioning system (GPS) data (modified from UNAVCO). Within the eastern Basin and Range Province, east-west crustal extension is accommodated along north-south striking normal faults. Within the western Basin and Range, a combination of normal faults and strike-slip faults accommodate northeast-southwest extension. This pattern of oblique extension reflects the increasing influence of the right-lateral transform fault associated with the San Andreas fault system between the Pacific and North American plates.
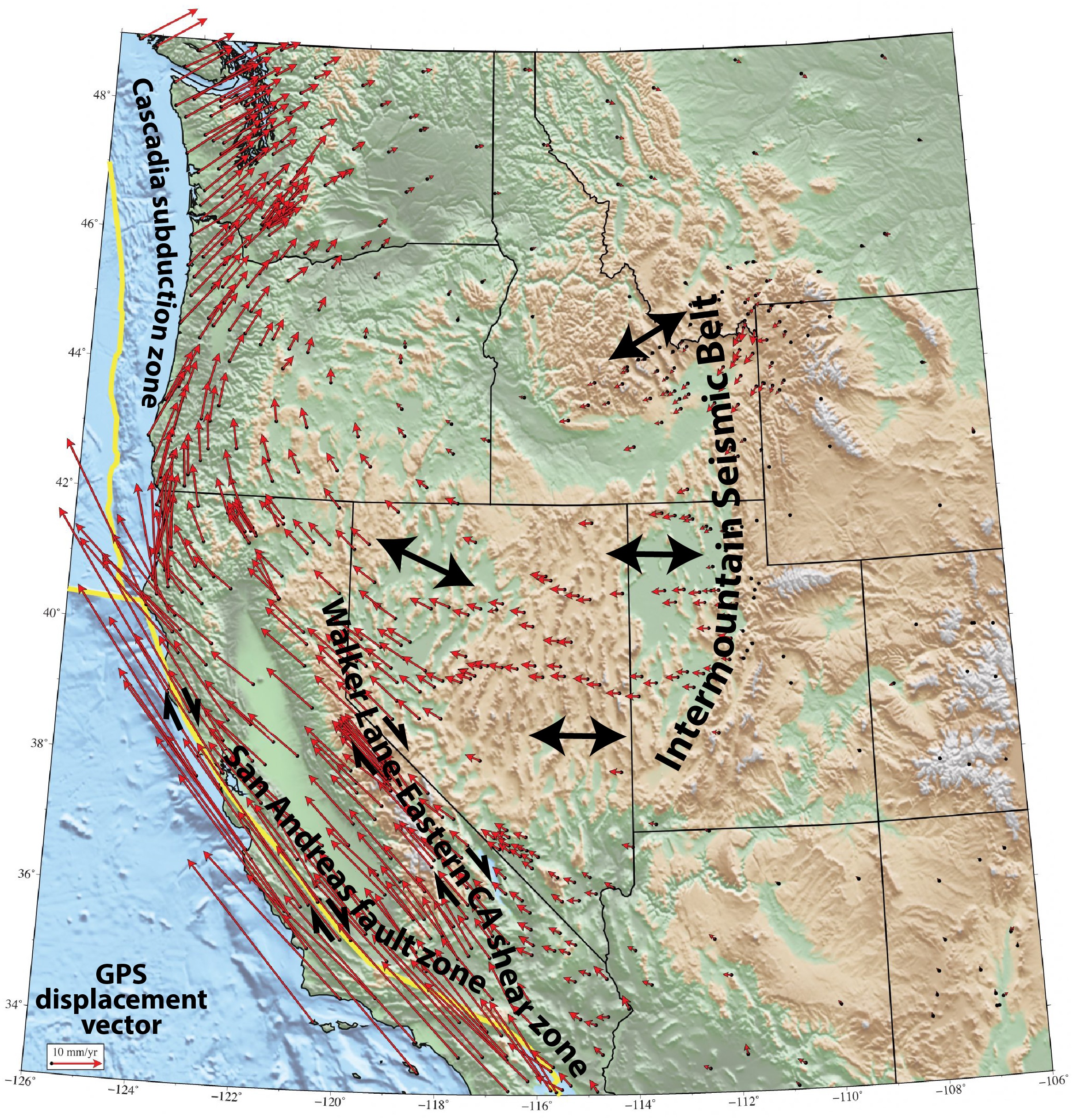
Basin and Range extension in Idaho
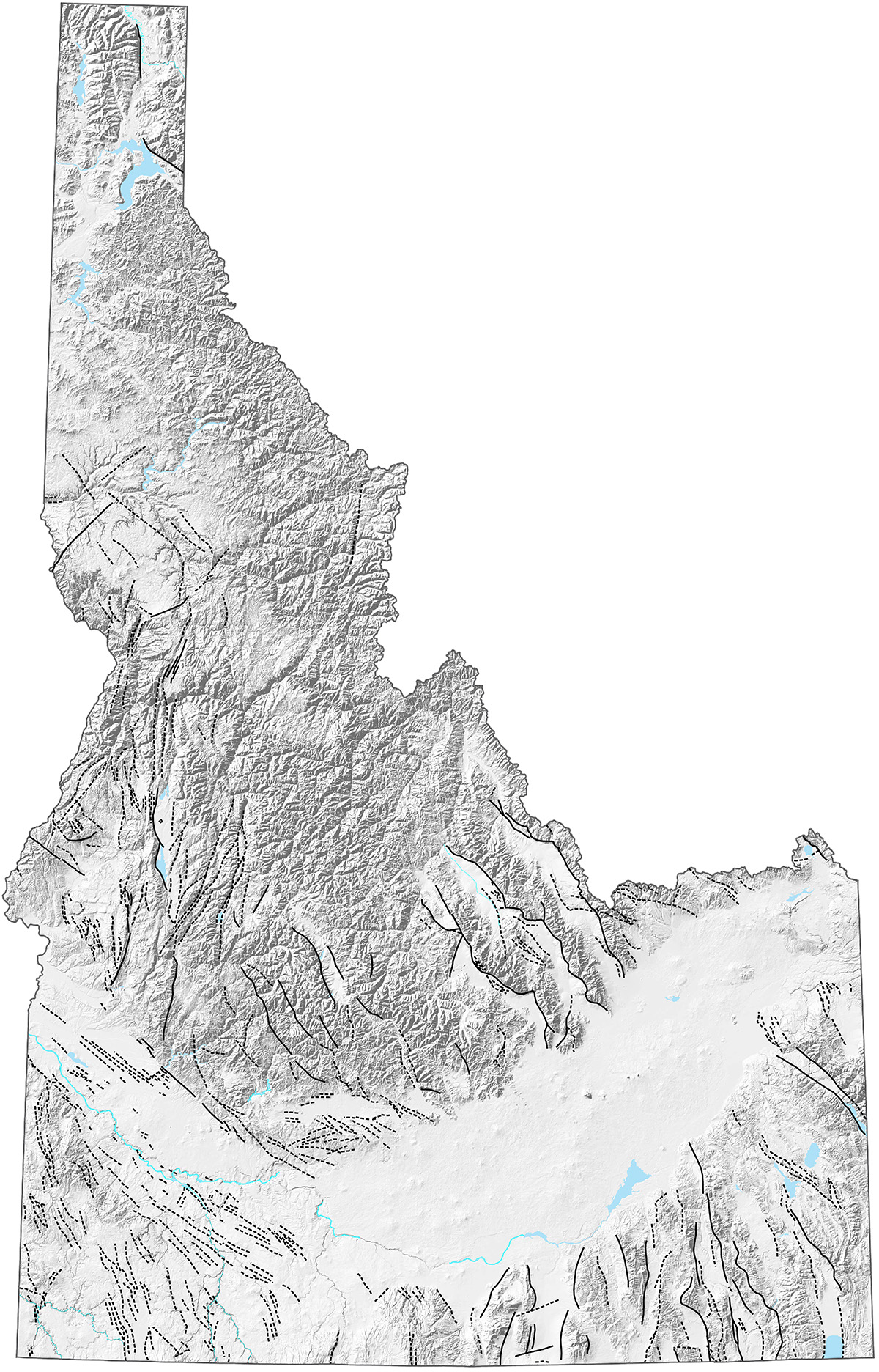
(Above) Map of normal faults in Idaho with fault displacement known to be younger than 16 Ma (black lines) (faults from Breckenridge et al., 2003). Soild lines are well-located and dashed lines are approximately located.
Idaho, Wyoming, and Montana host the northern limit of Basin and Range faulting. Many of the modern mountain ranges and basins in Idaho were formed by recent normal faulting, which has dropped down the basins and resulted in relative uplift of the adjacent mountain ranges. Some of these normal faults generate earthquakes when one side of a fault slips past the other. The most seismically active normal faults occur in southeastern Idaho and east-central Idaho within a broad region of late Cenozoic crustal extension called the Intermountain Seismic Belt, which is part of the Basin and Range province.
Most researchers do not consider the Yellowstone-eastern Snake River Plain volcanic system to have significantly affected development of the Basin and Range province. However, workers in the northern Rocky Mountains of Idaho, Wyoming, and Montana have documented enhanced rates of normal faulting that coincide with adjacent rhyolitic volcanism associated with the Yellowstone-eastern Snake River Plain volcanic system (e.g., Rodgers et al., 2002; Pierce and Morgan, 2009).
(Above) High rates of normal faulting in the northern Basin and Range Province of Idaho, Wyoming, and Montana coincide temporally with rhyolitic volcanism associated with the Yellowstone-eastern Snake River Plain volcanic system (modified from Rodgers et al., 2002). This relationship suggests that magmatism weakens the lithosphere and results in faster rates of upper crustal extension in this part of the Basin and Range.
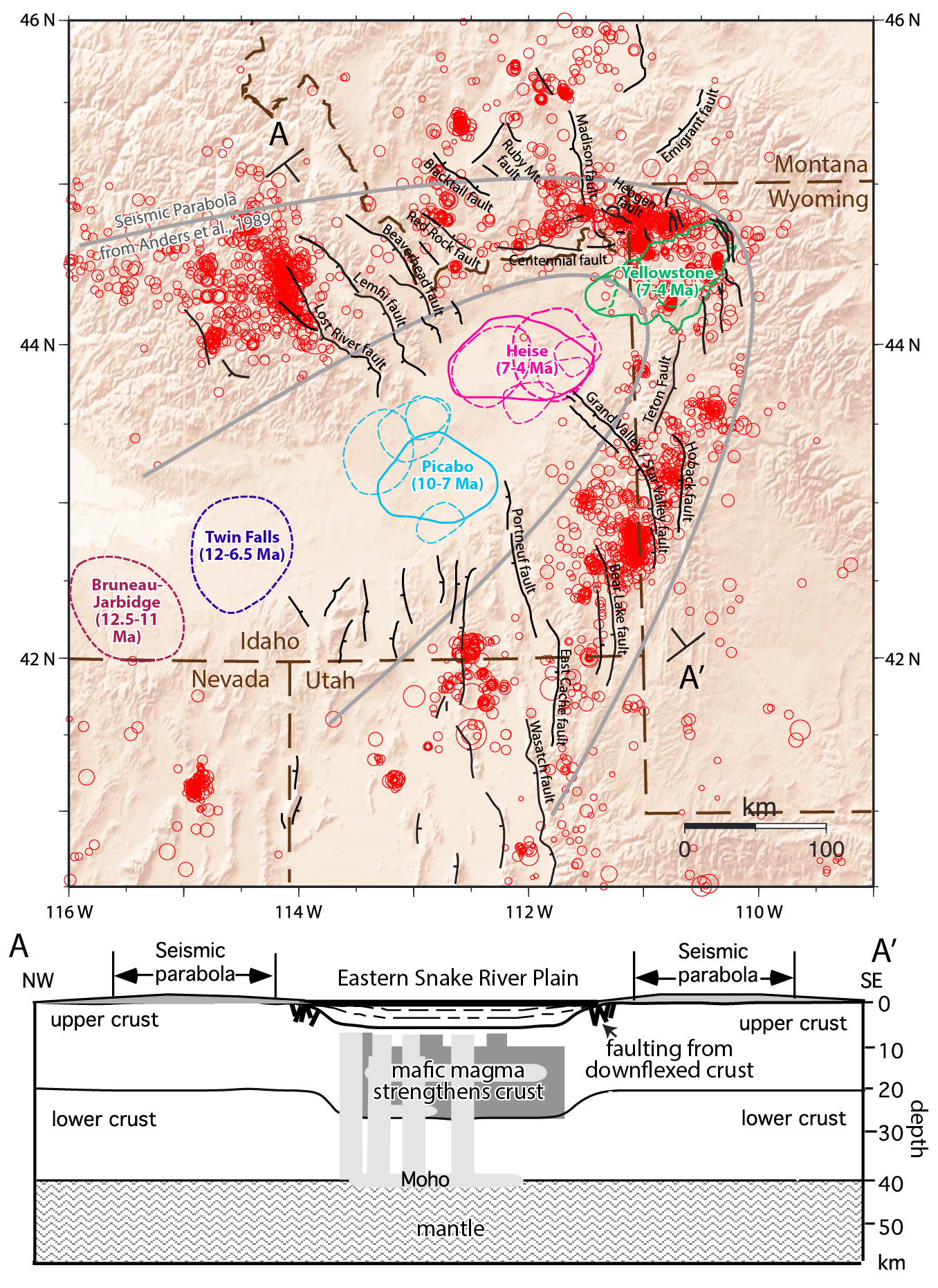
Though rhyolitic volcanism associated with the Yellowstone hot spot appears to have enhanced normal faulting within the adjacent Basin and Range, the eastern Snake River Plain, which is now buried beneath basalts, is a region of very little seismicity. Thus, the pattern of earthquake epicenters in the northern Rockies defines an arcuate shape, with its apex at Yellowstone and the region within the eastern Snake River Plain within the internal part of this "seismic parabola" (Anders et al., 1989). Researchers in the region hypothesize that the lack of seismicity within the eastern Snake River Plain is a result of emplacement of a thick, mid-crustal sill of mafic rock that accompanied Yellowstone volcanism (e.g., McCurry et al., 2009). Because mafic rocks are stronger than felsic rocks, this may have strengthened the crust.
(Left/Above) (Upper figure) map of northern Basin and Range Province (modified from Anders et al., 2014). Red circles show historic earthquakes (larger circles are larger earthquakes) and generalized limits of seismicity ("seismic parabola" of Anders et al., 1989), colored polygons are rhyolitic volcanic centers associated with the Yellowstone-Snake River Plain volcanic track, and black lines are normal faults of the northern Basin and Range. (Lower figure) Schematic cross section--with approximate location shown in upper map--showing hypothesized mafic sill and downflexed crust beneath the eastern Snake River Plain (modified from Rodgers et al., 2002).
Basin and Range extension in Idaho
Continue to Module 10 - Columbia River basalts
References and further reading
Anders, M.H., Geissman, J.W., Piety, L.A., and Sullivan, J.T., 1989, Parabolic distribution of circumeastern Snake River Plain seismicity and latest Quaternary faulting: Migratory pattern and association with the Yellowstone hotspot: Journal of Geophysical Research: Solid Earth, v. 94, p. 1589–1621.
Anders, M.H., Rodgers, D.W., Hemming, S.R., Saltzman, J., DiVenere, V.J., Hagstrum, J.T., Embree, G.F., and Walter, R.C., 2014, A fixed sublithospheric source for the late Neogene track of the Yellowstone hotspot: Implications of the Heise and Picabo volcanic fields: Journal of Geophysical Research: Solid Earth, v. 119, p. 2871–2906.
Bennett, R.A., Wernicke, B.P., Niemi, N.A., Friedrich, A.M., and Davis, J.L., 2003, Contemporary strain rates in the northern Basin and Range province from GPS data: Tectonics, v. 22.
Breckenridge, R.M., Lewis, R.S., Adema, G.W., and Weisz, D.W., 2003, Miocene and younger faults in Idaho: Idaho Geological Survey Bulletin.
Dewey, J.F., 1988, Extensional collapse of orogens: Tectonics, v. 7, p. 1123–1139.
Dickinson, W.R., 2006, Geotectonic evolution of the Great Basin: Geosphere, v. 2, p. 353.
Faulds, J.E., and Henry, C.D., 2008, Tectonic influences on the spatial and temporal evolution of the Walker Lane: An incipient transform fault along the evolving Pacific–North American plate boundary, in Spencer, J.E., and Titley, S.R., eds., Ores and orogenesis: Circum- Pacific tectonics, geologic evolution, and ore deposits: Arizona Geological Society Digest, v. 22, p. 437–470.
Faulds, J.E., and Varga, R.J., 1998, The role of accommodation zones and transfer zones in the regional segmentation of extended terranes: Geological Society of America Special Paper, v. 323, p. 1–45.
Kapp, P., Taylor, M., Stockli, D., and Ding, L., 2008, Development of active low-angle normal fault systems during orogenic collapse: Insight from Tibet: Geology, v. 36, p. 7–10.
Leeder, M. R., and R. L. Gawthorpe, 1987, Sedimentary models for extensional tilt-block/half-graben basins: Geological Society, London, Special Publications, v. 28, no. 1, p. 139-152.
McFadden, R.R., Mulch, A., Teyssier, C., and Heizler, M., 2015, Eocene extension and meteoric fluid flow in the Wildhorse detachment, Pioneer metamorphic core complex, Idaho: Lithosphere, v. 7, p. 355–366.
Pierce, K.L., and Morgan, L.A., 2009, Is the track of the Yellowstone hotspot driven by a deep mantle plume? — Review of volcanism, faulting, and uplift in light of new data: Journal of Volcanology and Geothermal Research, v. 188, p. 1–25.
Rodgers, D.W., Ore, H.T., Bobo, R.T., McQuarrie, N., and Zentner, N., 2002, Extension and subsidence of the eastern Snake River Plain, Idaho: Tectonic and Magmatic Evolution of the Snake River Plain Volcanic Province: Idaho Geological Survey Bulletin, v. 30, p. 121–155.
Vogl, J.J., Foster, D.A., Fanning, C.M., Kent, K.A., Rodgers, D.W., and Diedesch, T., 2012, Timing of extension in the Pioneer metamorphic core complex with implications for the spatial-temporal pattern of Cenozoic extension and exhumation in the northern U.S. Cordillera: Tectonics, v. 31, p. TC1008.
Credits
This page was originally created by Paul Link, Idaho State University; it was modified and updated by Dave Pearson at Idaho State University.